Energy transition: Which technologies matter?
The call to keep all technology options open is often a sign of helplessness or shameless patronage politics. A better understanding of technological disruptions becomes a prerequisite for democracy.
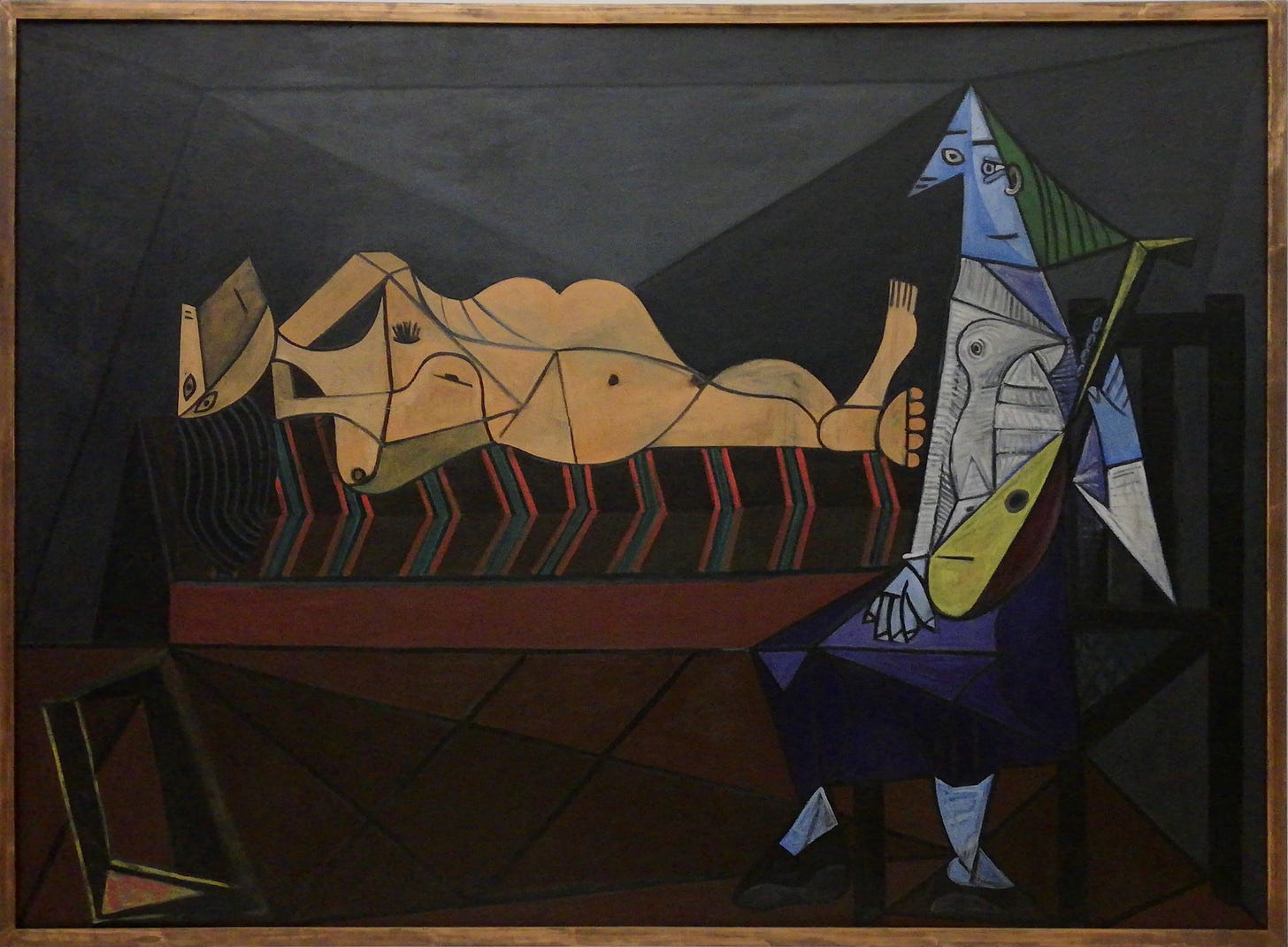
Translation from the German original by Dr Wolfgang Hager
Especially as regards energy policy, there is a lot of chatter about technologies and innovations. Politicians, journalists and interested citizens often have a surprisingly accurate knowledge of technical details, but hardly understand the principles behind them. That is why they are often helplessly at the mercy of the opinion-mongering of interest groups. The call for technology openness without questioning the framework conditions is often an admission of dangerous incompetence or shameless patronage politics.
Traditional fossil energy technology has led us into the climate crisis
Some historical context can be helpful here. Since the beginning of industrialization, some two hundred years ago, the earth's population has grown from one to eight billion people. Since the 1960s, that growth rate has been declining — due to rising prosperity, not catastrophes. The increase has been made possible by technology and rising energy consumption. In 1800, humanity still relied entirely on traditional biomass and muscle power. Then people started to rely mainly on fossil fuels: first coal, then oil, and later natural gas. From 1900 to 2020, fossil fuel consumption increased 22-fold. The highest growth occurred between 1960 and 1980. This growth, however, was extremely unevenly distributed: per capita, the U.S. consumed sixty times more than Indians in 1965, but just nine times as much in 2022, “only” doubling its consumption.
All the basic technologies for supplying energy in the year 2000 were already known by 1900. In the ensuing century, they were merely somewhat refined: oil and natural gas supplemented coal, and all machines became a bit more efficient. One remained in the familiar world of classical physics. New scientific discoveries essentially served to stabilize the established structures. When extractable resources became scarce in the 1970s, the oil industry used new material science, geological and drilling methods to develop new deposits. U.S. President Reagan halted his predecessor Carter's efforts to increase reliance on renewable energy. Fracking caused oil prices to fall again. Fossil fuel growth continued, even though it was known that this could not end well.
It was the growth of fossil fuel consumption which changed the nature of the problem: the limiting factor was no longer the accessible deposits of fossil resources, but the capacity of the climate system to absorb the combustion gases. Since 1956, there have been increasingly vocal warnings about the climate consequences of rising fossil fuel consumption, yet this has increased six-fold since then. Despite intensive international climate research and diplomatic negotiations, today, a good thirty years after the Kyoto agreement, we are in a seemingly hopeless situation. In just a few decades, we have used up most of the buffer capacity of the climate system.
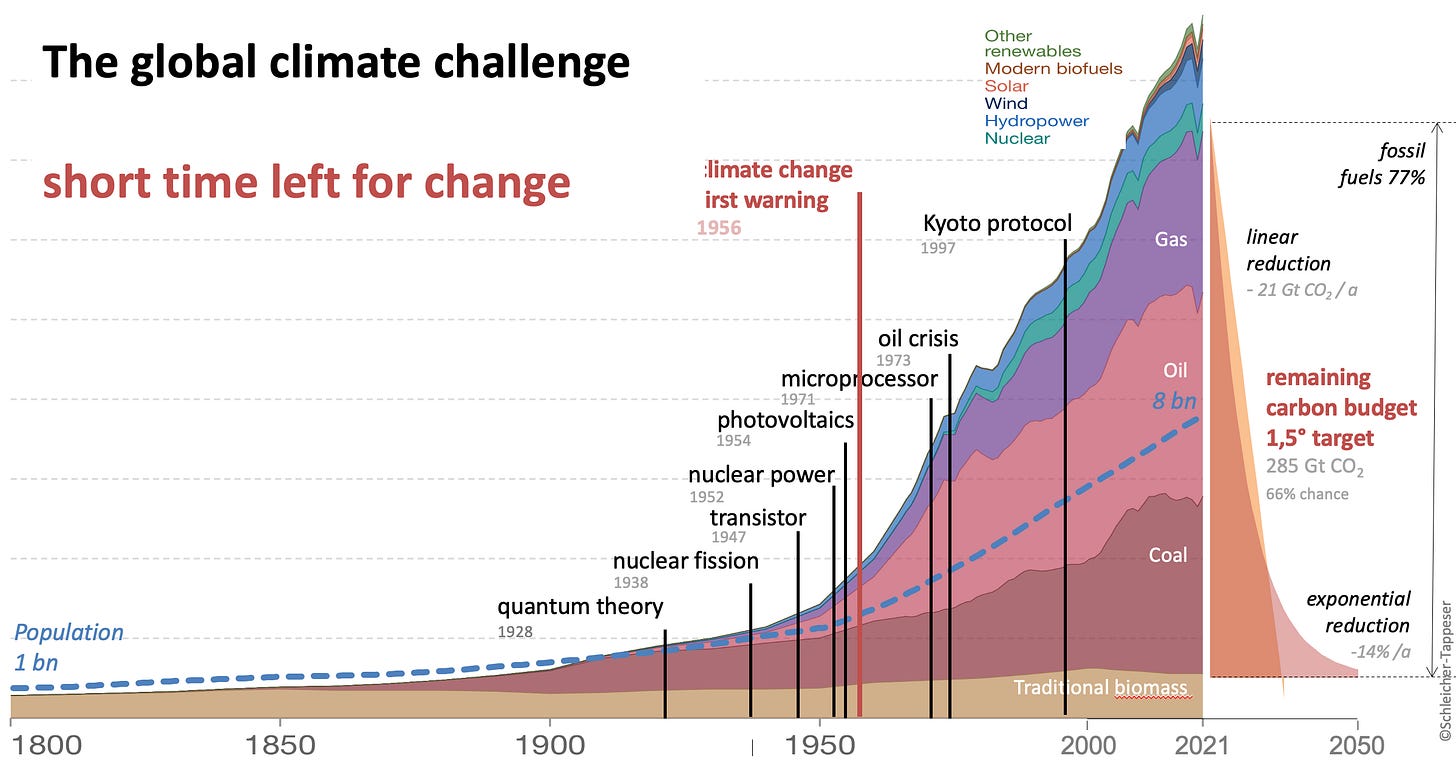
We have only a few years left to completely transform the entire energy supply. With the current efforts to gradually "decarbonize" the existing supply system, this will not work.
Seen in this light, the story of the energy system and living conditions on Earth looks almost hopeless. And many young people despair. But one can also consider the developments of the last century from a different perspective:
The discovery of nanoworlds
About a century before we reach a limit with our traditional energy technology and disregarding it threatens to destroy the foundations of our civilization, natural science already came up against limits of the classical scientific view of the world. But public perceptions of technology remain wedded to this classical view to this day.
Classical physics, which was in harmony with our everyday sensual experiences and had allowed to build very effective machines and instruments, came up against its limitations when studying radiation. On the level of atoms, confusing phenomena were observed, which no longer matched our everyday experiences. After more than twenty years of troubling findings, a convincing mathematical formulation for relationships that seemed to defy common sense was finally found in 1926-1928 with quantum theory. This affected not only theoretical physics, but had an impact on all sciences dealing with very small structures. Here, where it is not a question of meters but of nanometers, where everything can no longer be divided up again and again, nature takes leaps that are unfamiliar to us and which, only with the statistics of large numbers, result in the laws of nature we are familiar with. The first practical application was the atomic bomb, which showed people the far-reaching implications of the new insights.
After World War II, building on these revolutionary physics, applied technology also entered this world of atoms and molecules, a world that defies our imagination. Increasingly sophisticated measuring instruments made it possible to explore these nanoworlds. In one field of technology after another, advances suddenly became possible that dwarfed anything that had existed before in technical progress. In some cases, it took decades for basic discoveries to become technically usable.
We are most familiar with the development of computer technology, which illustrates the potential of the new nanosciences: the transistor was invented in 1947, the microprocessor in 1971, which then enabled software development largely independent of the hardware, all the way to artificial intelligence. But for that, solid-state physics first had to learn how to manufacture, harness and nanostructure extremely pure semiconductor crystals. Today, it allows 100 billion transistors to be accommodated on a chip. Microelectronics and computer technology advanced relatively quickly because they opened up huge new business opportunities.
Another example is biotechnology: in 1953, researchers succeeded in decoding the structure of DNA, in which life on earth stores its basic information. More than digitization, this involves risky interventions in evolution. It was only with the Corona vaccine, which saved millions of lives, that many people became aware of the incredible potential these technologies offer.
Great hopes were placed in atomic energy, the first attempt to use the new physical knowledge to build a major new industry. In thermal power plants, heat from nuclear fission was to replace coal-fired boilers. From the beginning, its close association with the development of nuclear weapons won atomic energy enormous financial resources but also staunch opposition. Ultimately, it failed because of two fundamental risks: The effort required to permanently shield radioactive radiation and to control what was in principle an explosive chain reaction became too great as knowledge of the dangers increased. The impact on energy supply remained modest; after 75 years of development, nuclear energy supplies only 3.7% of the world's primary energy.
Billions of people have learned to use one or another of these new technologies, especially all kinds of computers. But they have largely given up trying to understand the technology. It is no longer comprehensible to the senses. Discussions of technology tend to focus on the disruptive developments in computers, digitization, artificial intelligence. In the process, we lose sight of the extent to which our advance into the nanoworlds is now also speeding up developments in other fields of technology dramatically, linking up separate fields and placing traditional technologies in a new overall context. It is only since the turn of the millennium that the profound upheaval triggered by the physics revolution a century ago has become widely manifest.
The use of elementary processes at the level of atoms and molecules allows, first, a compression and acceleration of processes and, second, an undreamed-of variety of links that are not possible or explorable at the macro level. In many areas, it is only the new information technology that has made it possible to tap into this diversity — for example, in the mathematical modelling of chemical compounds and crystal structures. Therefore, nanoscience-based technologies have a development potential and a development speed that far outstrip classical technologies - as long as they are not dead ends.
For our energy system, I see four nanoscience-based technology clusters that today are driving the energy transition forward at breakneck speed. For a long time, these were neglected because the energy industry resisted structural changes. Now, however, they have passed a point of no return, not only technically but also economically, which is driving their implementation ever faster.
Four nanoscientific innovations drive the energy transition
Photovoltaics — the first of the four fundamental nanoscience innovations for the energy transition — had to prevail against far greater opposition than nuclear energy. Invented in the 1940s, it was used in space exploration from the late 1950s, but was still extremely expensive. Development progressed slowly, as the entrenched industries of electricity, coal, oil and gas took at most a passing interest. It was not until the environmental movement in Germany around the turn of the millennium and then especially China after 2010 that the breakthrough came. With a steep cost reduction, PV has now overtaken all other methods of power generation. As costs continue to fall, photovoltaics will become the dominant energy source of the future. However, it has a handicap: generation depends on sunshine — so it relies on other technologies to compensate.
Power electronics, in my view, is the second decisive innovation for the energy transition — and completely underestimated by the general public. Without it, highly efficient variable-speed motors and wind turbines, modern electric cars and robots, flexible automatic control of plants and an increasingly flexible power grid would be inconceivable. It was only slowly developed from electronics focused on processing signals. Even for large loads, it can now be used to convert electrical currents of all kinds into each other almost at will. And because it is closely related to signal electronics, power electronics can be remotely controlled digitally, without any human or mechanical mediation. After a long period of scant interest from the electrical industry, solar and wind power are now making these conversion options attractive.
In power electronics, too, nanoscience is now ensuring speeds of development that would have been unthinkable in conventional technologies: Since 1995, power density has increased a thousandfold. By significantly increasing the efficiency and automated control of all energy transformations involving electricity, power electronics increases the flexibility of the energy system many times over, thus facilitating the integration of volatile solar and wind power.
The third decisive innovation is new batteries. Only a detailed understanding of the intercalation of ions in crystal structures and systematic research into new materials makes it possible to store electricity ever more cost-effectively in ever more powerful and lighter batteries. The learning curves are now even steeper than in photovoltaics. The increasing energy density allows ever more diverse mobile applications up to small aeroplanes. Decreasing costs and increasing reliability make their use in power grids more and more interesting to improve grid utilization, security of supply and integration of solar power. The rapidly developing field of physical-chemical electricity storage is vast and promises further dramatic advances:
A wide range of chemical systems for closed battery cells with increasingly sophisticated nanotechnology structures,
Flow batteries, where separation of converter and storage of "charged" liquids promises lower costs for longer storage times
And - much less efficiently - improved electrolysers and fuel cells for long-term storage with hydrogen.
New conversion technologies turning electricity into radiation are the fourth element of my quartet of fundamental nano-scientific innovations pulling forward the energy transition: semiconductor-based light-emitting diodes, lasers, microwaves, etc... These enable huge gains in energy efficiency. Lighting, material processing, process heat and photochemistry are of particular importance:
The massive efficiency gains in lighting made possible by the widespread introduction of light-emitting diodes over the last decade are well known.
New methods of materials processing are only just beginning to emerge. Drilling, cutting and welding with lasers have developed rather slowly. Increasingly rapid advances in laser technology, control technology, power electronics and nano-material science are now leading to the rapid development of 3D printing, promising strongly reduced material, transport and energy needs.
Process heat at high temperatures can be provided ever more easily, specifically and economically with microwaves, magnetic induction or lasers - based on electricity, and precisely digitally controlled.
At the dentist, we can marvel at the progress of photochemistry when illumination turns soft pastes into high-strength dental fillings in just a few seconds. High-precision targeted energy delivery for chemical reactions can replace energy-guzzling thermal processes while avoiding unwanted byproducts. The potential is huge, but requires major conversions - which is why the development of suitable processes and catalysts is in many cases only just beginning.
These four areas of innovation have overcome crucial economic and technical hurdles after long stalling by established industries. In their interplay, they enable a much more sustainable and cost-effective energy system. Direct generation of electricity from sunlight, a highly flexible electricity system, and highly efficient electrical applications all play crucial roles. In my series of articles on the history of energy technology, I present this development in more detail - both in its scientific-technical and its social dimension.
The explosion of technological possibilities is an opportunity to put the brakes on climate change
Never before in history has there been such a rapid and profound expansion of technological possibilities as in the past twenty years. Measured in terms of the gains in efficiency, the cost reductions of new energy sources, and the diversity of new materials and processes, the current fireworks of innovations and successful implementations leave the previous industrial revolutions far behind - and these are only the energy-relevant aspects.
That's good news for the moment. Because the unprecedented escalation of technical-economic possibilities offers the chance to end the escalation of man-made climate change. Even with the technologies now available, it is possible to put the supply of energy we use and the way we use it on a different track in a short period of time.
However, each new technological opportunity poses difficult questions for our societies. The fundamental transformation of energy supply and use touches on deeply embedded structures and habits of our industrial societies and the international order. The two central questions are:
What will be the design of the new system?
Will the change be fast enough to avoid ecological tipping points due to climate change?
The political framework of the energy system — with its market regulations, standards, privileges and subsidies — has grown for two centuries with legacy energy technology and its powerful industries. These framework conditions must now be reshaped in a hurry. It must be clear to everyone that this cannot be done without clashes of interest. We need to learn about the options now open for shaping the future, create visions for a different future, engage in informed debate — and very quickly overcome old structures and habits. This challenge is unique in its urgency in industrial history. It requires unusual efforts. Talk about keeping all technology options open throws sand in our eyes. We still have opportunities to shape a future worth living. So let's use them.