Nanoscience has made electricity directly from sunlight unbeatably cheap
Episode 10 of the series: History of technology - The discovery of nano-worlds enables a renewable energy supply for all
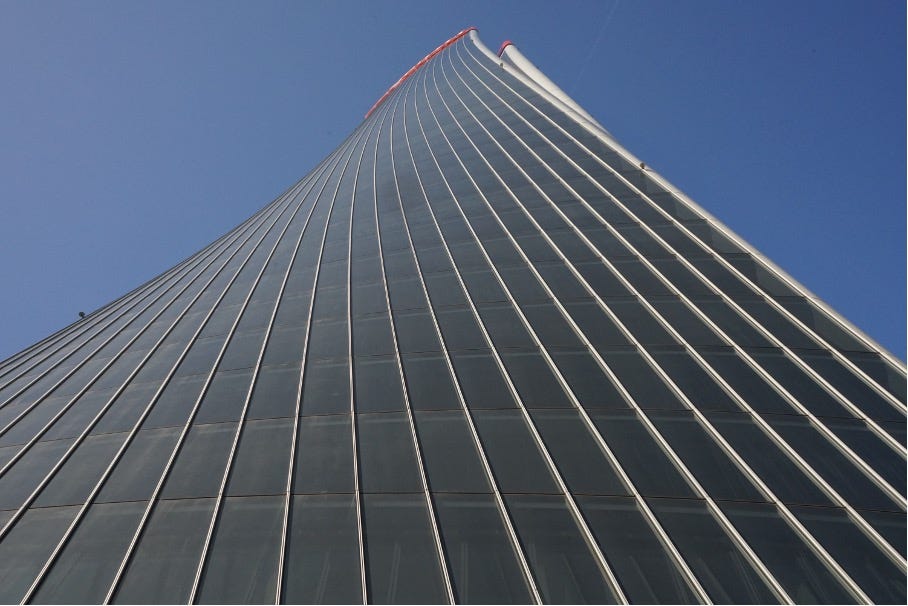
Translation from the German original by Dr Wolfgang Hager
We only have a few years to replace fossil fuels as the basis of our industrial civilisation. This requires a radical transformation of our energy systems. Photovoltaics, now the cheapest way to generate electricity, will be at the heart of this process. It is the latest consequence of a profound change in the scientific worldview that has opened the door to previously unknown nanoworlds on the scale of atoms.
Solar radiation, which includes ultraviolet and infrared radiation in addition to the visible light spectrum, provides almost all the energy we need - only atomic energy and geothermal energy have other origins. Solar radiation has a wide variety of effects:
Solar radiation makes plants grow, which convert it into chemical energy.
Solar radiation heats up the bodies it hits. It warms the earth's surface, causes water to evaporate and thus drives the weather.
Sun’s rays can be concentrated with mirrors and lenses, yielding very high temperatures where they hit bodies.
Dark surfaces absorb solar radiation better than light ones, leading to different surface temperatures.
In greenhouses and similar heat traps, solar radiation penetrates through glass, but heat radiation cannot escape, heating up the space behind the glass well above ambient temperature.
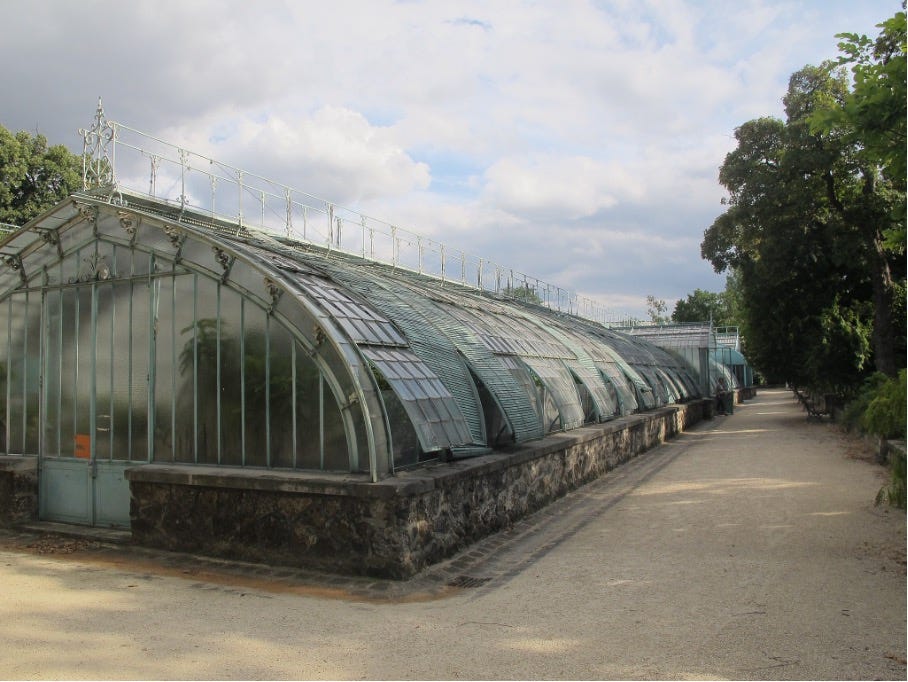
What caused these phenomena, however, could not be explained for a long time. And even more mysteries were added in the 19th century. Until quantum theory, developed between 1900 and 1930, made it possible to describe more precisely what happens when radiation encounters matter. The description had to be given at the nano-scale of atoms and molecules, where other laws apply than in our everyday macro-world. These theories then allowed the breakthrough to a direct conversion of sunlight into electricity, through what we now call photovoltaics. No more conversion detours, and high efficiency. But one after the other.
Semiconductor researchers discover a promising method
The development of photovoltaics began with Alexandre Edmond Becquerel, who discovered in 1839 that the illumination of metal electrodes in conductive liquids could release charged particles. In 1876, William Grylls Adams showed that it was indeed possible to generate electricity by exposing selenium to light. In 1883, Charles Fritts built the first photovoltaic module from selenium cells. However, it could not be used for practical power generation due to its low efficiency.
Since such phenomena could not be explained by the physics of the time, a number of scientists set out to investigate these weak and often disputed effects more closely. Finally, in 1905, Albert Einstein, in order to explain the external photoelectric effect first discovered by Becquerel, put forward the light quantum hypothesis for which he was awarded the Nobel Prize. It states that light consists of discrete energy packets, the quanta. He subsequently developed the special theory of relativity, which postulates, among other things, the equivalence of mass and energy.
Already in 1900, Max Planck had assumed that thermal radiation is portioned into quanta - but he did not initially want to accept the same for light. It took many more doubts, discussions and experiments until the 1920s, when the view became generally accepted that radiation can be described both as a wave (energy) and as a particle (matter), which are mutually exclusive in our everyday experience. With the wave model, it was possible to explain how light rays could be refracted in a lens and thus focused. With the particle model, one could explain how a light quantum, also called a photon, can knock an electron out of its atomic orbit and thus release electrical energy. With the mathematical formulation of quantum theory, a convincing representation of the interaction between energy and matter was found between 1926 and 1928. It became the basis of the new material sciences. It does not provide a vivid description, but provides a mathematical explication that works in practice (see episode 1 of this series).
In view of the energy yield of selenium cells of less than one percent of the irradiated sunlight, their technical use remained limited for many decades to measuring instruments such as light meters for photography. In 1940, Russell S. Ohl, who had been experimenting with silicon for diodes at Bell Laboratories since the 1920s, discovered quite by chance that the transition between differently doped silicon crystals (i.e. crystals containing different kinds of foreign atoms) generated electricity when exposed to light - much more efficiently than selenium. Strictly speaking, this was not exactly the same effect that Becquerel had discovered at the time. There, the light quanta had knocked electrons out of a metal surface; here, the process took place between different semiconductor layers.
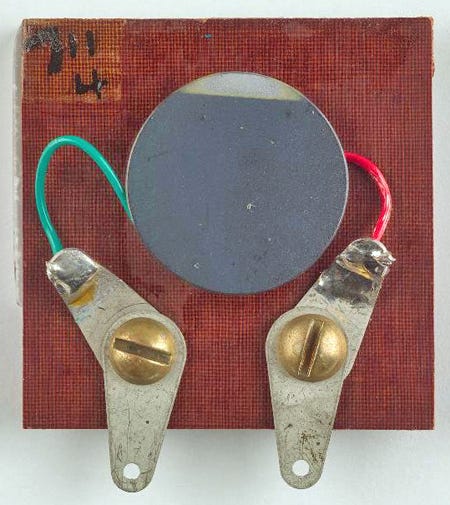
It was not until 1950 that William B. Shockley, who had co-invented the transistor in 1947 (see episode 5), was able to provide a (quantum) theoretical description of the processes involved in the irradiation of silicon boundary layers. The technique of producing high-purity silicon crystals and doping them over a wide area, which was developed for the production of transistors, then brought about the breakthrough.
In 1954, Chapin, Fuller and Pearson of Bell Laboratories were able to present to the public a two-square-centimetre silicon cell with an efficiency of 6%. That may not sound like much, but it was already much more efficient than the energy yield of plant photosynthesis (see episode 6). From the solar radiation on a given surface, the first silicon cell could produce five to ten times more electrical energy than a plant can convert into fuel (chemical energy). Thus, two years after the first electricity generation with atomic energy, photovoltaics was born as a serious source of electricity.
Politics and oil prices derail photovoltaics again and again
Photovoltaics initially remained extremely expensive. This is because an adequate area of silicon was needed to capture sunlight, and it had to be of consistent quality. From 1958, American space satellites were equipped with them. PV cells proved to be a durable, reliable source of energy. Space travel developed into a business segment from which a small photovoltaic industry could earn a living and further develop the technology.
But the influential nuclear industry had no interest in seeing photovoltaics develop here on Earth: At the beginning of the oil crisis in 1973, the chairman of the American Atomic Energy Commission, on behalf of President Nixon, presented a major report on the energy future of the US. It recommended a five-year energy research budget that included 4 billion for nuclear energy, 1.5 billion for fusion energy and only 36 million for photovoltaics, which were not even mentioned in the action programme. This was in blatant contradiction to the internal proposal of the National Science Foundation, which had collaborated on the report and estimated that with research funding of 250 million, photovoltaics could become competitive by the year 1990, and subsequently supply over seven percent of American electricity generation with steep growth rates by the year 2000.
It was not until the mid-1970s that more photovoltaic power was used for terrestrial applications than in space travel, after it had been possible to reduce costs significantly. This was achieved, among other things, by using silicon waste from the semiconductor industry as a starting material. Radio applications in remote areas kicked off the process. Then all the major oil companies began to equip their unmanned oil platforms with photovoltaic systems. Buoys and remote lighthouses for shipping were also equipped with them.
Under the impression of the first oil crisis, Japan and Germany had launched special programmes to promote photovoltaic research in 1974 and 1977. From 1977 onwards, the newly elected President Carter, in contrast to his predecessor, focused on determined research promotion, as well as targeted development of growing niche markets. With increasing production volumes, things progressed quickly at first.
In autumn 1980, I had the opportunity to report in detail on the third European Photovoltaic Conference in Cannes: Paul Maycock of the American Department of Energy under President Carter predicted prices of 5 to 9 US cents per kilowatt hour of solar electricity from the roof of one's own house as early as 1986. The European participants were not so optimistic, but they, too, reckoned that this could be achieved by the beginning of the 1990s. Oil companies, in particular, had invested in photovoltaics and threatened to squeeze the electricity industry with a new kind of decentralised power source that would have challenged their business model. By 1980, oil was scarce and the price of oil, barely recovered from the first oil crisis in 1973, had quadrupled again, peaking at $40 per barrel ($141 in today's prices) (see episode 8).
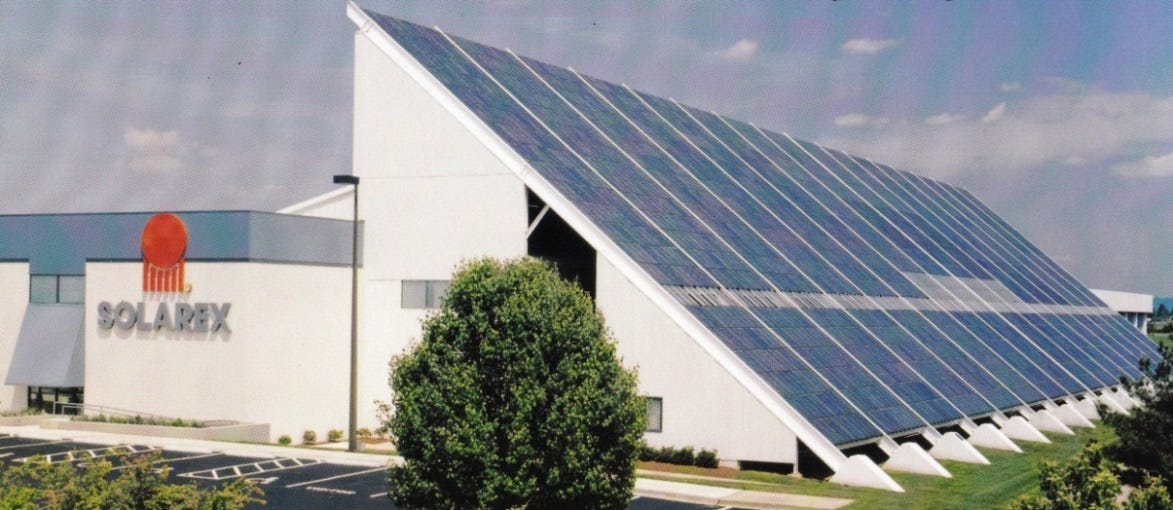
However, photovoltaics did not take off that quickly; people had been too optimistic. The two successive oil price shocks led on the one hand to a worldwide recession and on the other hand to a more sparing use of energy. In the USA, the energy intensity of the national economy (energy consumption per unit of gross domestic product) fell by 21% between 1980 and 1990. As a result, oil prices and oil production plummeted and oil companies lost interest in photovoltaics. In addition, from the nineties onwards, new drilling technologies ensured the development of deposits that were previously inaccessible (see episode 8) - this dispelled the oil industry's worries about the end of the oil age. The oil price did not rise again until after 2000.
The political wind also changed when Ronald Reagan took over the US presidency in 1981. He drastically cut back public support for renewables. Environmental concerns slipped down the political agenda, after, still under Carter, the comprehensive “Global 2000” report had just caused a worldwide sensation.
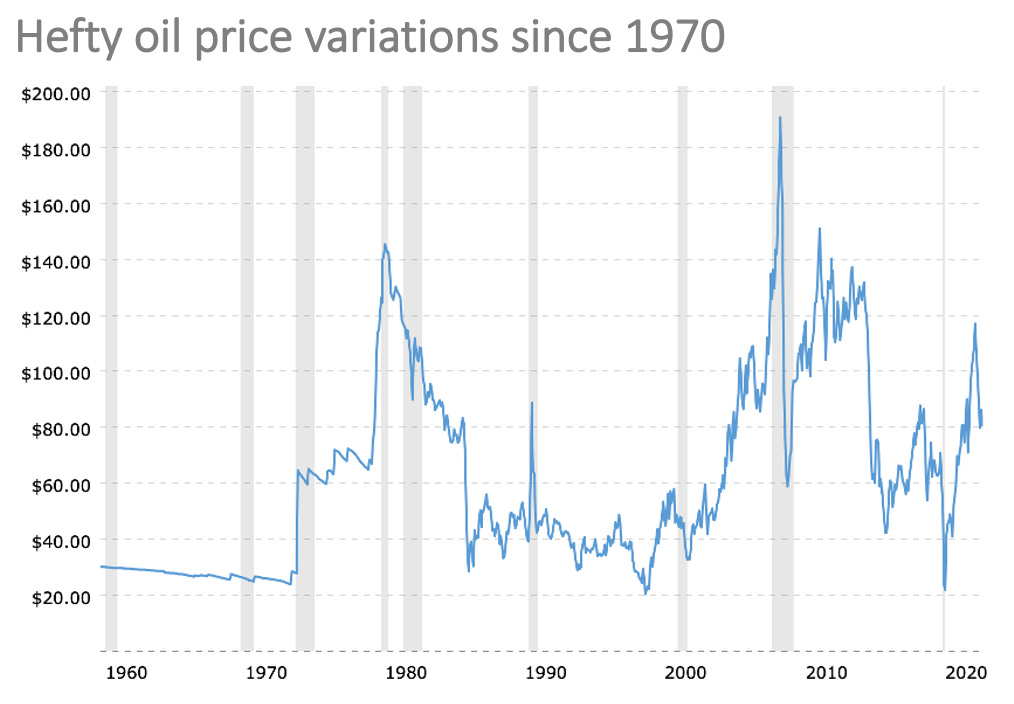
The cost reduction in photovoltaics nevertheless continued. Between 1980 and 1990, the price dropped to about a quarter. In 1982, the first 1 MW plant was put into operation in California. In the nineties, more companies entered the solar market. In 1994, Japan announced a 70,000-roof programme and became the international leader in PV activities. From 1993 to 2001, Bill Clinton and his vice-president Al Gore took over the presidency in the USA and again committed themselves to photovoltaics. In 1997, the USA launched a one-million-roof programme.
Two recommendable podcasts for anyone interested in new technologies that can help in the climate crisis:
Cleaning Up - Leadership in an Age of Climate Change. Host: Michael Liebreich. On
Apple, on
Spotify.
Redefining Energy. Hosts: Laurent Segalen + Gerard Reid. On
Apple, on
Spotify.
In 1998, the first Red-Green coalition came to power in Germany. Especially the Green Ministry for the Environment made a strong case for renewable energies against considerable opposition. The Renewable Energy Act (EEG) passed in 2000 triggered the development of a rapidly growing market for photovoltaics: there was a guaranteed feed-in tariff for solar power to the grid - a model that was later adopted in many countries. At the end of 2000, about 1500 MWp (megawatt peak, the output at maximum solar irradiation) were installed worldwide, 12% of them in Europe. Ten years later (2010) there were 27 times more, and Europe had a share of 74%. Germany alone accounted for 45% of the world's installed capacity. In 2011, almost 8000 megawatts of photovoltaic capacity (MWp) were newly installed in Germany. This level has not been reached again, as the growth of the German solar market was politically choked off. Between 2011 and 2014, 107,000 jobs were lost in the German solar industry. What had happened?
The electricity industry strikes back
Due to the unexpectedly strong price reductions, it became apparent from 2010 that photovoltaics would soon become competitive in some market segments and challenge established market structures.
By 2012, self-generated electricity had become cheaper for private individuals in Germany than electricity from the grid. Photovoltaic electricity production by private investors began to erode the sales of the established electricity suppliers: in 2012, almost eighty percent of photovoltaic capacity was installed on roofs in Germany. In the EU, the share was only slightly lower. Installed PV capacity grew at impressive annual rates between 2007 and 2010: 54% in Germany, 68% in Europe and 50% worldwide. During the same period, the price of solar modules dropped by 21% annually. This began to seriously worry the big utilities. Even worse than the declining market shares was the impact of solar on the most profitable time segment. Solar was strongest precisely during the consumption peaks around noon, where premium prices could be charged. The share prices of the largest electricity suppliers halved (2009-2011: RWE - 60%, EON - 43%).
Under massive pressure from the electricity industry and citing the rising surcharges for the feed-in tariff, conservative governments in Europe's most important markets put the brakes on photovoltaics within a very short time by introducing a series of new rules. Feed-in tariffs were lowered in rapid succession. The market collapsed to 15% of 2011 volumes in Germany, less than 3% in Italy and 31% in Europe overall.
Yet continued PV growth with adequate compensation for consumers would have been beneficial even in the short term: Between 2011 and 2018, the reduction in the electricity price due to the feed-in of wind and solar power exceeded the costs of the surcharge by 45%.
The rise of China
There was a second reason for the collapse of the Western photovoltaic markets: Chinese producers had begun to conquer the market for PV modules and the long-established Western industries that had supplied the equipment for the conventional system showed little interest in committing themselves to the new technology.
In the mid-1980s, the first two small production lines for solar cells were installed in China. After the UN Conference on Sustainable Development in Rio in 1992, the official "Agenda for 21st Century China" called for priority to be given to renewable energy in the national development strategy. In 2001, China had installed just over 1% of the world's photovoltaic capacity (24 MWp) and produced 5 MWp modules. Except for special applications in remote areas, photovoltaics were too expensive for China. But then China took advantage of the fast-growing German market in particular to build up its own PV industry, was quick to learn and ramped up the production of cells and modules with annual growth rates of over 100%.
In 2007, China overtook Japan and Germany and for the first time produced more than 1000 MWp of cells and modules, more than 98% of which were exported. The domestic market was very cautious: at that time only 100 MWp had been installed and a strategy paper of the State Planning Commission foresaw only 1800 MWp installed capacity for 2020. In 2010, over 12,000 MWp were produced and still 96% were exported - but in view of the lower prices and the discussions in Europe, China was preparing to develop its own market. From 2009 onwards, domestic installations more than doubled every year. In 2011, a feed-in tariff was introduced. In 2014, 28 gigawatts, seventy times as much capacity had been installed as five years earlier. Nevertheless, far more than half of the production was still exported.
In 2020, 36% of the world's photovoltaic installations were in China and the target set in 2007 for that year was exceeded by a factor of 140.
Today, China's global market share in all stages of the PV module manufacturing value chain is around 80%. Since 2011, China has invested ten times more than Europe in PV production. For a long time, Europe supplied the production machinery. Today, the ten largest manufacturers of production equipment are also Chinese. Photovoltaics have become an important export product for China, with an annual volume of over 30 billion dollars. Chinese investments in PV production have now also become an important economic driver in neighbouring countries such as Vietnam or Malaysia.
The cheapest energy source after 40 years of cost reduction
This massive expansion of production and intense intra-Chinese competition have helped to bring costs down ever further: Within forty years, the price of photovoltaic modules fell by more than 99%. From 2010 to 2021 alone by around 90%.
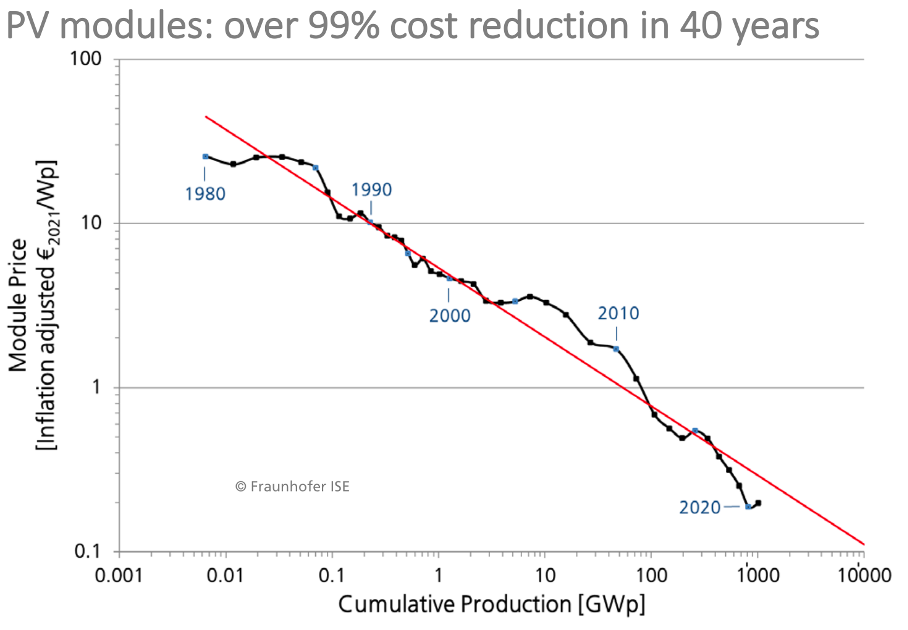
Photovoltaics has thus become the cheapest technology for generating electricity. This is now also being said by institutions that were previously quite sceptical. At an average of 17% per year since 2010, the cost of the electricity generated has not fallen quite as fast as the module costs. This is because a photovoltaic power plant consists not only of modules, but also of other components whose price falls much more slowly. For smaller plants in particular, other investment costs are now higher than the modules themselves.
Every year, the global investment bank Lazard provides the most cited comparison of the electricity production costs with different technologies. According to Lazard, at 13 to 20 US cents per kilowatt hour, electricity from new nuclear power plants is considerably more expensive than from photovoltaic power plants at 2.8 to 4.1 US cents. Even for already existing and fully amortised nuclear power plants, Lazard calculates with 2.9 ¢/kWh.
With this cost dynamic, Photovoltaics is also leaving other renewable power generation technologies behind .Between 2010 and 2021, the average cost of electricity from photovoltaics fell by 88%, compared to 58% for offshore wind and 67% for onshore wind.
Where do we go from here?
Now that it is recognised as the cheapest technology for electricity generation, it seems inevitable that photovoltaics will play a central role in the future global energy supply. However, how this highly fluctuating source of electricity can be integrated into a comprehensive supply system can only be clarified when looking at the system as a whole, and against the background of other energy technology innovations.
Before looking at these, however, I would like to explore the potential and consequences of photovoltaics in more detail in the next instalment of this series. First, there is the question of whether the rapid cost reduction can continue. Secondly, we will ask whether photovoltaics are actually superior - economically and in terms of a sustainable energy system - to other renewable power generation technologies that have grown less quickly. And finally, it is interesting to see whether and how a larger role for photovoltaics could shift power relations between industries, institutions and even countries. On these questions, the history of photovoltaics can provide important clues. For this, it will also be necessary to take a closer look at these technologies.
Very good read. The basic point that people miss is the lack of climate awareness in the masses. Hopefully things are changing but it is getting too late as such. https://bit.ly/3UKAfDe